IBC-16-19
KEYWORDS: Bridge pile encasement, confinement, fiber reinforced polymer (FRP), underwater pile jacket, alkalisilica reaction (ASR), asset management, prestressed concrete, rehabilitation, maintenance, Barron River bridge
ABSTRACT
Octagonal PSC piles in a bridge over the crocodile-infested Barron River near Cairns in tropical north Queensland, Australia, experienced severe ASR cracking and required remediation to protect against corrosion damage. Forty piles were repaired underwater by encasing in a protective jacket consisting of thin glass FRP laminate sheets wrapped around the piles to create a seamless, impervious, cylindrical shell subsequently filled with a low viscosity resin that sealed the concrete and filled any voids and cracks.
INTRODUCTION
The bridge on the southbound carriageway of the Captain Cook Highway over the crocodile-infested, tidal estuary of the Barron River 4.5 miles (7km) north of Cairns in tropical north Queensland, Australia, was constructed in 1977.
The bridge is 579 feet (176.5m) long and 34 feet (10.4m) wide, with seven spans of 77 feet (23.5m). The superstructure consists of prestressed concrete (PSC) beams and reinforced concrete (RC) deck, while the substructure consists of piled abutments and blade piers rising from pile caps, each supported on 10 closely-spaced PSC piles.
Piles are 20.7 inches (525mm) octagonal PSC piles. All piles are raked with clear spacing between piles as low as 12 inches (300mm).
The top of the pile cap is nominally at mid-tide. Tidal range is around 15 feet (4.5m) and the upper 4 feet (1.25m) of piles is within the tidal range. However, the piles are generally underwater and during normal tidal movements, it is rare for more than 20 inches (0.5m) to be exposed (Fig. 1).
In 2000, severe ASR cracking was identified in 13 of the 40 PSC piles in the four piers located in the main river channel.
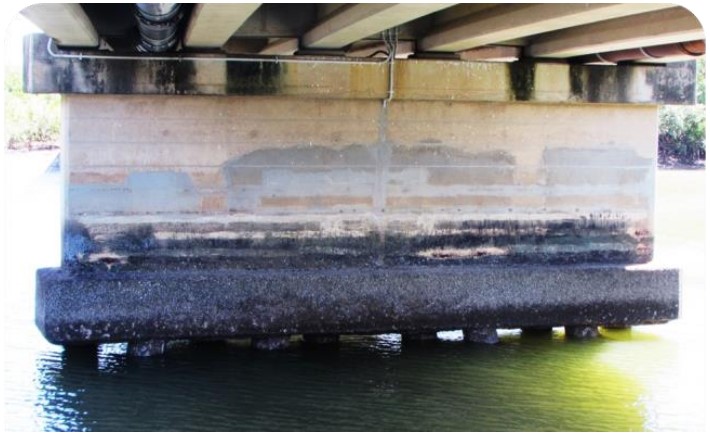
To protect the piles from premature failure due to corrosion, and to inhibit the development of ASR cracking, the state road authority – the Queensland Department of Transport and Main Roads (DTMR) – determined that encasing the piles was the preferred long-term treatment.
This paper describes a number of phases associated with a 15-year journey in search of a suitable, economical pile encasement solution, and describes details of the adopted solution and its installation.
EARLY CONSIDERATIONS
Initial preference was to use a traditional RC jacket to protect the piles. However, further investigation identified a number of site challenges to traditional encasement solutions, including:
The close-spacing of piles precluded the use of individual RC encasements and increased the complexity and cost of an RC encasement considerably.
Having utilized Fiber Reinforced Polymer (FRP) jackets in above-water pile remediation works, DTMR was interested in exploring the feasibility of utilizing FRP jackets in underwater environments. Sample of FRP jackets that were common for pile encasement projects are shown in Fig. 2. There are several inherent problems with these products:
- The vertical seam along the side of the jacket allows ingress of moisture and oxygen so the corrosion process will continue;
- The vertical seam and bolts introduce a plane of weakness in the jacket that limit the confining pressure exerted by the jacket to the swelling pile; and
- The jackets must be manufactured for a specific size; this delays project scheduling and construction time
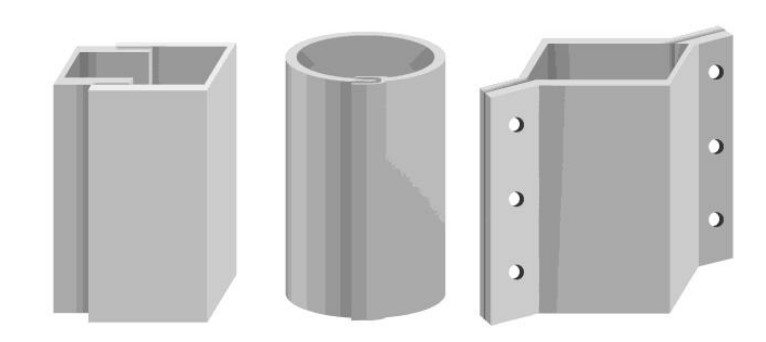
of FRP Jackets
FRP PROTYPE DEVELOPMENT (2003)
A review of available FRP jackets failed to identify a commercially-available jacket that met the desired technical requirements and could be easily and confidently installed underwater.
DTMR became an active partner with the University of Southern Queensland (USQ) and the NSW Roads and Traffic Authority (RTA) in a joint project to develop a prototype FRP jacket for concrete piles suffering ASR cracking.
Various researchers, including Carse (1992), had shown that the rate of ASR reduced as internal pressure increased. One of the primary goals, therefore, was to develop an FRP jacket that would not simply act as a barrier to inhibit the ingress of chlorides, moisture and oxygen, but would provide sufficient confinement to allow a buildup in internal pressure to slow the ASR reaction. A practical operating pressure of 2MPa was adopted for purposes of design of a confinement system (Fig. 3). As discussed above, the FRP jackets that were in use at the time could not provide an impervious moisture barrier nor could they resist a confining pressure of 2 MPa (300 psi).
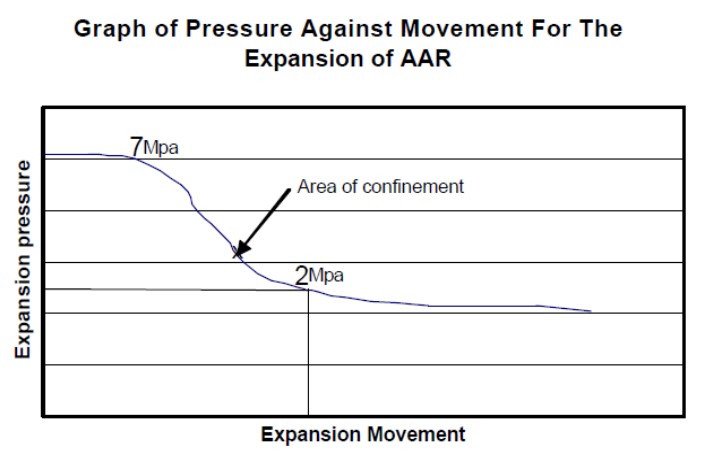
A prototype FRP jacket was subsequently designed and tested. At the time, it was considered that underwater-curing epoxies were not sufficiently advanced to meet the project objectives, and a mechanical jointing system was preferred. The prototype was designed with a finger joint system, with the fins being turned in to be anchored in the hardened grout subsequently placed in the annulus between the pile and jacket (Fig. 4).
Testing of the prototype was partially successful, proving the concept, but requiring further development to progress to a commercial product (McGuffin et al. 2003).
Unfortunately, the project did not progress beyond the prototype phase.
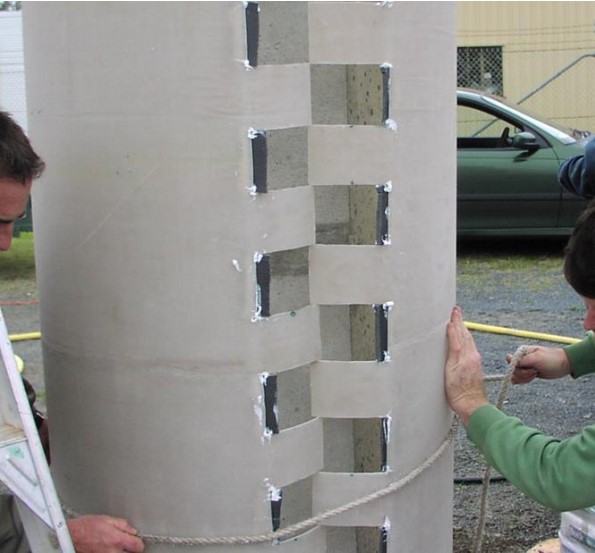
MARKET EXPLORATION (2006)
In late 2006, there was a call for Expressions of Interest (EOI) for the jacketing of the piles. Encouragement was given to offer innovative jacketing solutions including FRP jackets.
From four submissions, all proposing RC encasement, two companies were selected to develop and provide an indicative price for their proposed solutions.
Though one option initially appeared to offer significant cost savings, further development taking into account the specific challenges of the site, resulted in a final estimate well in excess of the available funding.
At this stage, it was decided to:
PROJECT REINITIATION (2014)
In 2014, all piles were cleaned and inspected by divers at a total cost of A$150,000 – 50% of which was for cleaning, 30% for installation of crocodile protection and 20% for inspection.
The underwater inspection indicated that the number of cracked piles had increased from 14 in 2004 to 22 in 2014.
At the same time, the recently developed PileMedic® system was identified as a pile remediation system that might be capable of meeting the technical and practical needs of this project at an economical cost (around 40% of the previously tendered prices for a traditional RC encasement).
A project proposal was prepared and subsequently ratified as a trial installation by DTMR’s Structures Branch. Finally, funding was secured, design details finalized and scheme documents prepared to enable the works to proceed.
DTMR’s in-house delivery arm (Roadtek) were engaged to undertake the works, sub-contracting QuakeWrap (Australia) to provide training and technical advice during the works and local commercial divers to undertake underwater works.
THE ADOPTED SOLUTION – PILEMEDIC®
Independent from these developments in Australia, a new form of FRP product was being created by one of the co-authors in the U.S. (Ehsani 2009). This development had its beginning dating some 25 years earlier when FRP products were being studied for strengthening of bridge piers to resist seismic loads (Saadatmanesh et al. 1992). The early generation of FRP was applied using a technique known as wet layup. In that system, fabrics of carbon or glass are saturated with epoxy and directly wrapped (i.e. bonded) to the column for increased confinement. While that system does provide structural enhancement, it has a few limitations. The surface of the column must be repaired and patched to bring it to a smooth surface prior to wrapping of fabric. Furthermore, wet layup systems require immediate bonding to the surface of the column and are difficult to implement when a column is submerged in water — as was the case here.
The new PileMedic® laminates are constructed with specially-designed equipment. Sheets of carbon or glass fabric up to 60 inches (1.5 m) wide are saturated with resin and passed through a press that applies uniform heat and pressure to produce the laminate (Figs. 5 and 6). The laminates offer three major advantages compared to other FRP laminates. First, by using a combination of unidirectional and/or biaxial fabrics, the laminate may provide strength in both longitudinal and transverse directions; this is a tremendous advantage that opens the door to strengthening of columns for both bending and confinement. Secondly, they are very thin; with a typical thickness of 0.025 inches (0.66 mm), they can be easily bent into any shape circle with a diameter of 12 inches (300 mm) or smaller (Fig. 6). Lastly, the number and pattern of the layers of fabrics can be adjusted to produce an endless array of customized products that can significantly save construction time and money.
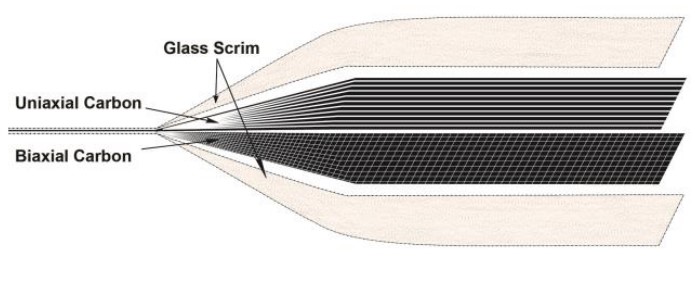
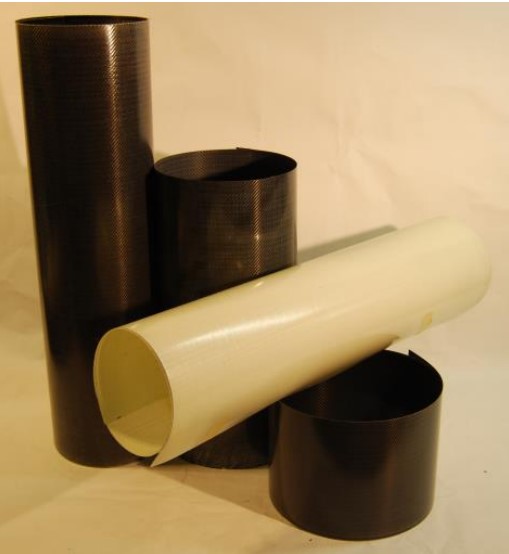
The fiberglass jackets depicted in Fig. 2 are basically a stay-in-place form for placement of grout. They offer virtually no structural benefit to the pile. For example, the tensile strength of some of the most popular fiberglass jackets is compared to PileMedic® laminates in Fig. 7.
The tensile strength of PileMedic® laminates ranges between 62 to 156 ksi (427-1080 MPa). The thickness of the laminates ranges from 0.01 to 0.03 inches (0.25 to 0.76 mm). It is noted that the development of an equipment to produce such uniformly thin and wide laminates is not a trivial matter and has been a major part of this product development.
The first application of PileMedic® for repair of submerged piles was carried out in Miami, Florida(Ehsani and Tipnis 2011). Details of the installation can be viewed in this video. Since that time, many transportation agencies have performed extensive testing of this product for various bridge pier applications. Among these are studies funded by Caltrans for concrete columns damaged in earthquakes (Yang et al 2015), Texas DOT for corroded steel H piling (Dawood et al 2015), and Nebraska Dept. of Roads for deteriorated timber piles (Mohammadi et al 2014). All of these studies have demonstrated the structural enhancement that can be obtained by using these laminates.
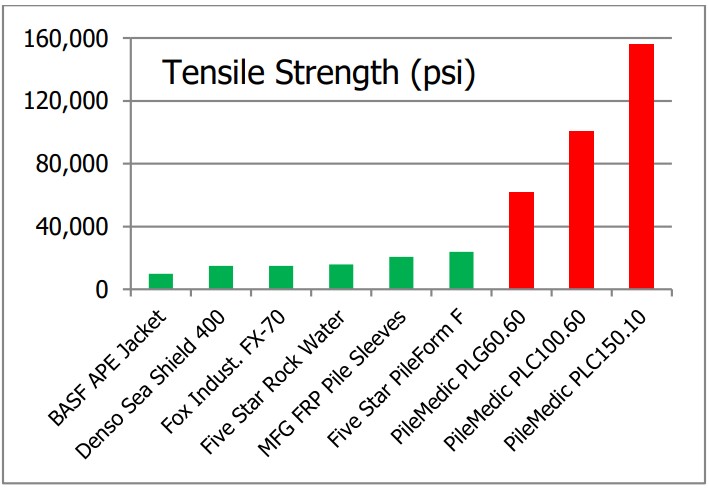
DESIGN OF THE PILE JACKET
When the objective of the jacket is to provide confinement for the column or pile, the design is explained with the aid of the free body diagram shown in Fig. 8. Assuming an element of the column with unit height, let us assume that the diameter of the jacket that encompasses the pile and the grout in the annular space is 25 inches (635 mm). If the jacket is made by wrapping two layers of PileMedic® PLC150.10 laminates, each one inch width of this laminate has a breaking strength of T= 4050 pounds (18 kN). The confining pressure, p, acting on the pile is the reaction of the sum of the 4 tension forces shown in Fig. 8, such that: 4T = p x Jacket Diameter, or p= 4T/Diameter = 4 x 4050/25= 648 psi (4.38 MPa).
As demonstrated, the laminates provide significant confining pressure for the pile. This confining pressure increases the compressive strength of the original pile and the newly placed grout. The amount of this confining pressure can be changed by using 0 40,000 80,000 120,000 160,000 Tensile Strength (psi) different types of laminates that have different tensile strengths, and by changing the number of layers that are wrapped around the pile. It is noted that this example is only intended to demonstrate the concept of the design. Safety factors and durability considerations must also be included. Design Guidelines such as those published by ACI Committee 440 provide detailed information for such factors.
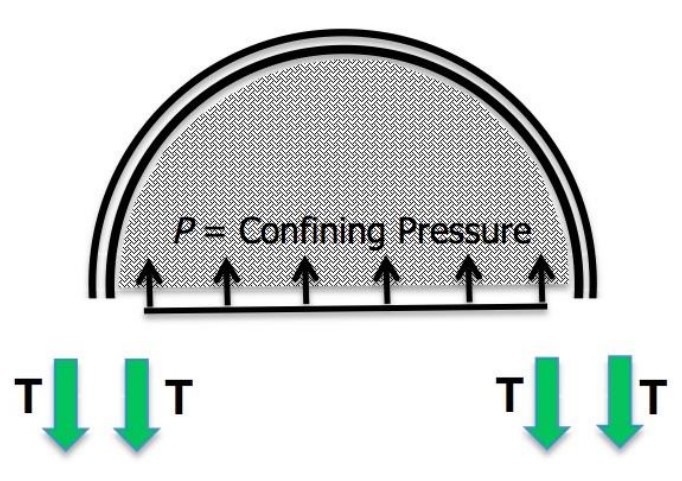
In some applications, the design may call for an increase in the bending capacity of the column as well. Laminates constructed with biaxial fabrics that provide strength in hoop and longitudinal direction (along the height of the pile) are ideal for such cases. By using an epoxy grout, the jacket will be bonded to the concrete pile and it will contribute to the bending capacity of the pile or column. The Caltrans test mentioned earlier was one such application.
FIELD INSTALLATION
A total of four piers each having 10 submerged piles were repaired on this project. The bridge site on Barron River is known for its crocodiles, bull sharks, jellyfish and the like. For safety of the diving crew, a steel cage was positioned around each group of pier (Fig. 9).
A barge was used to provide a flat working platform and a staging area for the materials and equipment (Fig. 10). The barge also included a small crane for loading of the materials such as the resin and grout.
PileMedic® laminates are supplied in rolls that are 4 feet (1.2 m) wide and 250 feet (76 m) long. A major advantage of this product is that the rolls can be easily cut to any length in the field, allowing a single roll of laminate to be used for repair of piles of virtually all shapes and sizes. Standard details call for the laminate to be wrapped twice (i.e. 720 degrees) around the pile with an additional 8 inch (200mm) extension beyond the starting point.
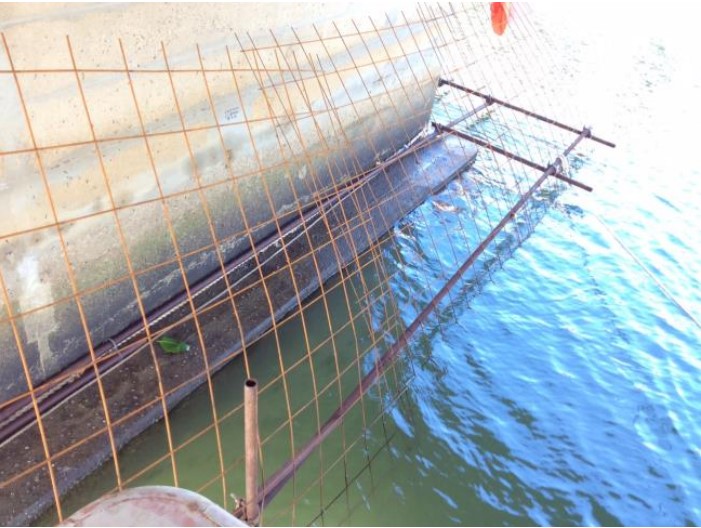
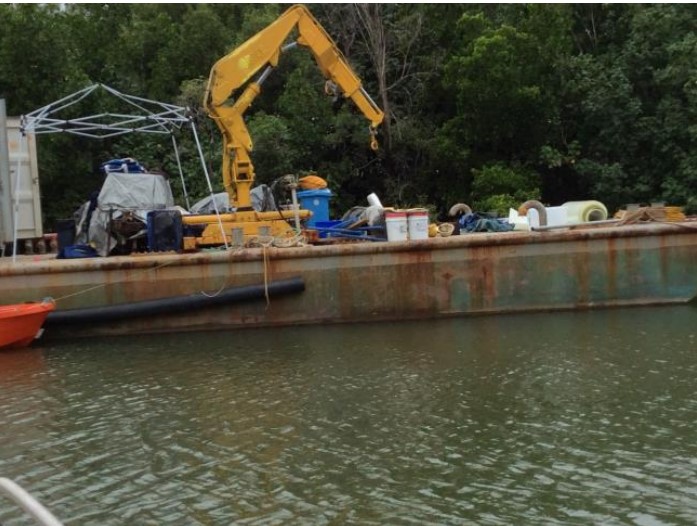
The repairs required creating a 2 inch (50mm) annular space around the piles to be filled with grout. This meant a diameter of 25 inches (635mm) for the jackets. Such jackets would be small enough to leave adequate working space between adjacent piles during the repair.
The circumference of such a jacket is 78.5 inches (2m). So, the 4-ft (1.2m) wide laminates were cut in lengths of 2×78.5+8 = 165 inches (4200mm) in the field. The laminate is placed flat on a table (Fig. 11). A two-part epoxy resin paste is supplied as a part of this system. This resin cures underwater and Figure 10 – Barge used as working platform eliminates the need for creating cofferdams and dewatering around the piles.
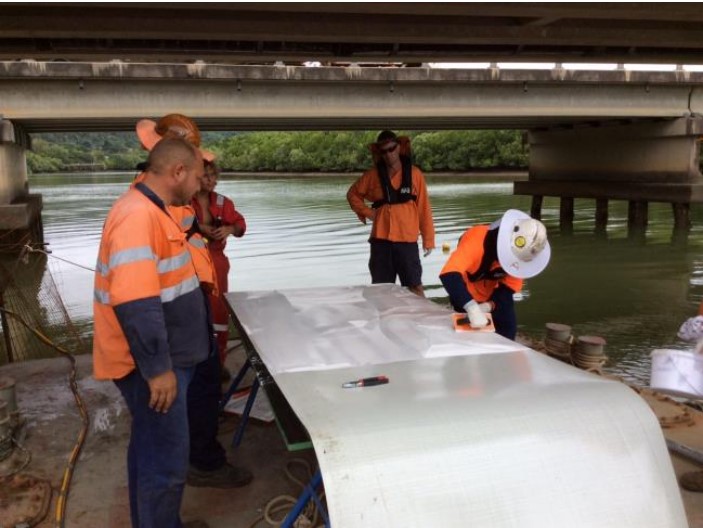
The laminate will be wrapped around the pile to create a two-ply shell. In doing so, the portion of the laminate that is adjacent to the shell does not have to be coated with epoxy. But the second ply and the 8 inch extension, must be coated. In this case, a length of 78.5+8=86.5 inches (2200mm) of the laminate had to be coated with epoxy. The twocomponent epoxy has a consistency similar to tooth paste and can be applied using a notched trowel to achieve a uniform thickness of 30-40 mil (0.75-1 mm).
Next, the coated laminate is picked up and passed over the steel cage to the diving crew (Fig. 12). In spite of their size, the lightweight jackets are fairly easy to handle once they are submerged. Spacers such as short pieces of 2 inch (50mm) diameter PVC pipe can be attached to the pile surface to define the required annular space for grout. The crew wraps the laminate around the pile over the spacers. The epoxy paste serves as a lubricant and allows easy adjustment of the size of the jacket. Ratchet straps can be wrapped around the shell to maintain its shape and dimension while the epoxy cures.
The first 4-ft (1.2m) tall jacket was installed at the base of each pile and extended 18 inches (450 mm) below the mud line. The next jacket was applied with a 4-inch (100 mm) overlap along the height of the pile. An epoxy paste is applied to this overlapping region. The process continued until a jacket of desired height was created. The diving crew consisted of 4 workers: 2 divers performing the installation, 1 back up diver and 1 supervisor. On the barge, a two-man crew was given the task of cutting the laminates and mixing and applying the epoxy paste.
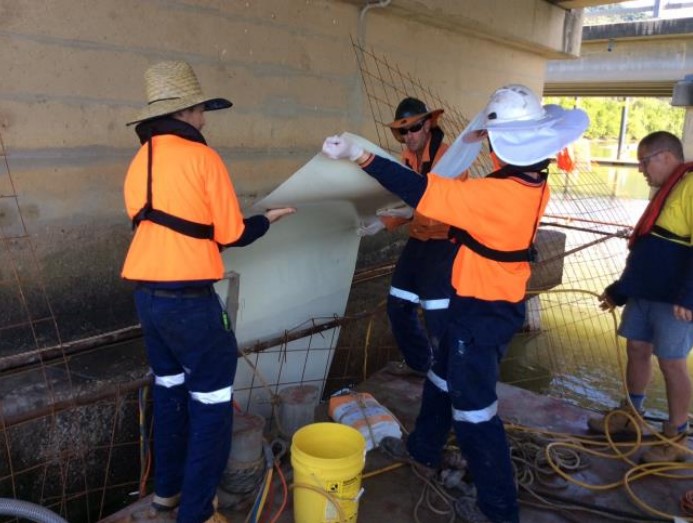
Once the jacket was installed, the annular space was filled with a cementitious underwater grout using the tremie placement method. A shear mixer and pump were utilized for this application. As the grout is placed, its hydrostatic pressure pushes the two layers of the laminate against each other for a perfect bond. Moreover, the heat of hydration of the grout can also assist with the faster curing of the epoxy paste. In the following day the ratchet straps will be removed and the repair is complete. Figure 13 shows the completed project after all 40 piles were successfully repaired.
The field installation had its unique challenges. The repairs were scheduled for the dry season to avoid flash floods in the river. The visibility in the murky water was low and often limited to 1 ft (300 mm). The large tidal flow ranging 6-10 feet (2-3 m) also had to be considered.
The project was completed in around 5 working weeks (35 days). This time was divide approximately into one day to install the steel protective cage, four days to wrap the piles and place the grout and one day to demobilize from one pier and move to the other. The crew performed the work exceptionally well, adapting to the constant challenges faced working in a trying tropical environment.
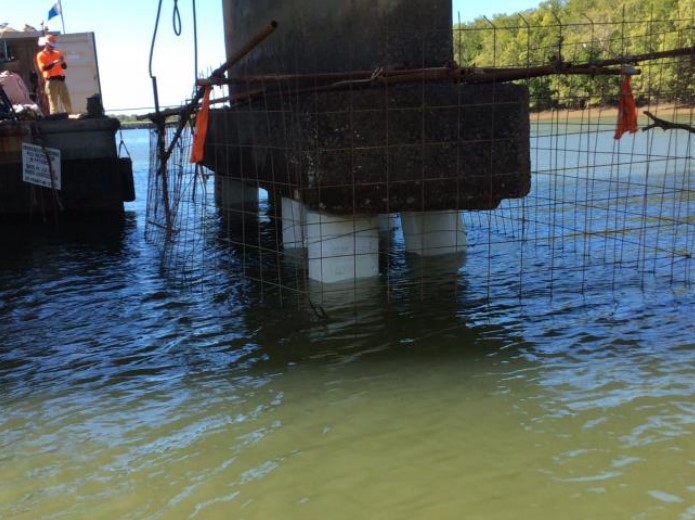
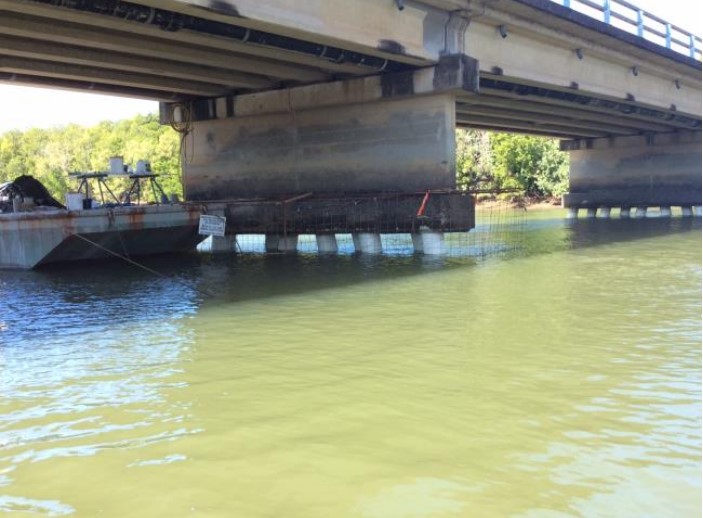
COST SAVINGS
According to the data from DTMR, the following cost data in Australian Dollars were reported. The original concrete encasement option in 2008 was bid at $2.0M which is equivalent to $2.46M in 2015. The PileMedic® option was estimated at $1.1M, but the actual cost ended up being only $0.95M. The $150,000 reduction was primarily due to ease of installation of the system and the efficiency of the diving crew as the project progressed.
The adopted solution resulted in savings of $1.5M or 160% compared to the original reinforced concrete encasement option. In retrospect, the agency’s delay of the project turned out to be a good decision as the development of this new technology resulted in significant cost savings.
SUMMARY AND CONCLUSIONS
The repair of 40 ASR contaminated submerged piles had lingered on for nearly 15 years. The options for a suitable repair to provide an impervious structural shell that would both stop the ingress of water and provide high confinement for the piles to resist the swelling of the concrete were limited at best. During this period a new product was developed and introduced to the market that could achieve both of these objectives.
The repairs were completed in 2015 with 160% cost savings compared to the original solution that was considered. This brought a happy ending to the 15 year search to solve this challenging problem.
ACKNOWLEDGEMENTS
This project was a truly collaborative effort that involved many agencies in two continents. The authors would like to thank the contributions of the Structures Branch and far North District of TMR, Roadtek as the General Contractor and JD Marine for underwater works.goo
The PileMedic® system and the method of repair presented are protected by U.S. Patent No. 8,650,831 and other pending U.S. and international patent applications.
REFERENCES
American Concrete Institute – Committee 440. Guide for the Design and Construction of Externally Bonded FRP Systems for Strengthening Concrete Structures, ACI 440.2R-08, ACI, Farmington Hills, MI, 2008.
Carse, A. Report on the mechanism causing cracking in the prestressed piles of the Houghton highway bridge, Queensland Department of Transport Technology Division, 1992.
Dawood, M., Karagah, H., and Shi, C. “Repair Systems for Deteriorated Bridge Piles Technical memorandum #4a,” TxDOT Project 0-6731, University of Houston, TX, 41 pp, May 2015.
Ehsani, M. and Tipnis, A. “Miami Building First to benefit from Innovative Pile Jacket,” Concrete Repair Bulletin, 16-18, July-August 2011. (Video)
Ehsani, M. “FRP Super Laminates Present Unparalleled Solutions to Old Problems,” Reinforced Plastics, 40-45, 53(6), 2009.
McGuffin, J., Heldt, T., Van Erp, G and Cattell, C. “Feasibility of a new pile rehabilitation system” Fibre Composites Design and Development, USQ Publication No FCDD-0300019_R3, 2003.
Mohammadi, A., Gull, J., Taghinezhad, R., and Azizinamini, A., “Assessment and Evaluation of Timber Piles Used in Nebraska for Retrofit and Rating,” Draft Final Report to Nebraska Department of Roads, Dept. of Civil Eng., Florida International U., Miami, FL, 51 pp, April 2014.
Saadatmanesh, H., Ehsani, M.R., and Li, M.W. “Seismic Strengthening of Concrete Columns with Fiber Composite Belts,” Proc., ASCE Materials Eng. Congress, Atlanta, August 1992, 677-690, 1992.
Yang, Y., L. Sneed, M. Saiidi, A. Belarbi, M. Ehsani, and R. He. 2015. “Emergency Repair of a RC Bridge Column with Fractured Bars using Externally Bonded Prefabricated thin CFRP Laminates and CFRP Strips,” Composite Structures, Elsevier, 727- 738, 133, 2015. (Video)