ABSTRACT
Steel frames utilizing Buckling Restrained Brace (BRB) elements have become popular in the last two decades. However, few practical solutions have been offered to retrofit braces in the large inventory of existing steel frames. These vulnerable non-seismic conforming concentrically braced frames pose a significant risk and challenge. This paper presents a new technique for retrofit of concentric braced frames using a recently-developed type of Fiber Reinforced Polymer (FRP) laminate. The lightweight laminates require no heavy equipment for installation, making the retrofit ideal for buildings with limited access. Results from preliminary large scale physical tests of the retrofit scheme are presented. The materials cost for the retrofit were less than $1000. The tests indicate the potential for a cost-effective, and easy-to-implement retrofit solution for the large inventory of seismically- vulnerable existing steel buildings
Introduction
Concentrically-braced frames (CBFs) comprise a significant portion of the national building inventory. The popularity of the CBF as a Lateral Force Resisting System (LFRS) stems from its economy through its high stiffness, requiring placement of braces in only a few bays of the overall floor plan at each floor. These bays can often be located along a stairwell, bathroom or elevator core, rendering the remainder of the floor plan open architecturally and designed economically for gravity load alone. CBFs are configured as single diagonals, chevrons, and single or two-story X-braces. Common CBF bracing elements for moderate forces include bolted double angles members that can “knife-fit the gusset, welded channels, tees, and in-cases, tension-only straps. For heavier loads, tubes or wide flange sections are used.
Steel CBFs are used extensively in buildings as a LFRS due to their high elastic stiffness and structural efficiency. However, the seismic performance of CBFs, which is dominated by the cyclic inelastic behavior of bracing members, is less robust than other common steel LFRS. The CBF brace elements act in both axial compression and tension, due to the cyclic nature of lateral forces (wind, earthquake), and thus the CBF brace element is susceptible to buckling in the earthquake.
Much research has been devoted to achieving higher levels of seismic performance in CBFs. As a result, modern braced-frame structures in regions of high seismic hazard are designed as Special Concentrically-Braced frames (SCBFs). SCBFs are designed for lower forces than required to remain elastic in the earthquake, and thus count on special detailing: compact sections for the brace member (e.g. tubes) to minimize the detrimental effects of instability, and carefully detailed connections to avoid fracture under tension. More recently, a high seismic solution gaining popularity is the Buckling Restrained Brace (BRB), which prevents buckling altogether.
Nevertheless, thousands of older non-compliant CBF structures still exist in regions of high seismic hazard, built in an era when seismic effects were not well understood, and thus prior to the creation of the stringent SCBF requirements. Further, in regions of low to moderate seismic hazard, in addition to older structures, most modern CBFs are designed without special seismic details, i.e. as Ordinary CBFs (OCBFs). These structures do not have the expected performance to survive a rare but destructive earthquake [1]. With the severe societal costs of rare but destructive Maximum Considered Earthquake (MCE) events [2], including the recent recognition of the increased risk for those events in regions of low to moderate seismicity [3], these structures represent a significant risk to the economy of the U.S. and safety of the general public.
The reason these vulnerable CBFs remain un-retrofitted in the U.S. building inventory, is due to the difficulty in achieving an economical yet effective retrofit for these structures. While retrofit solutions to provide ductile detailing is straightforward conceptually, the cost premium and practical limitations renders most retrofit schemes unworkable. In short, there are currently no both economical and effective retrofit techniques for low ductility CBFs [1]. This paper presents a recently-developed technique that allows economical retrofit of CBFs and some the preliminary test results.
Buckling-Restrained Braces (BRBs)
The BRB is a seismic bracing element that responds in ductile fashion to an earthquake. BRBs use an unbonded confining tube around a slender steel bracing core (Fig. 1a), thus allowing the core to yield in tension, but suppressing buckling in compression [4]. The typical BRB is: (1) a single piece yielding steel core with stiffer non-yielding end sections; (2) a restraining mechanism, often a steel tube with filled concrete with an end gap to permit permanent elongation. Under tension, the BRB behaves as a traditional steel brace; however, under compression, the yielding core is prevented from buckling by the restraining element, resulting in ductile, symmetric load behavior with high energy dissipation and compression strength than a CBF (See comparison in Fig. 1b). The BRB has been widely studied and is now commonly used for new construction of special seismic frames. The high-performing BRB is quite expensive ($25K/unit) [4].
BraceWrap® Retrofit Concept
The BraceWrap® retrofit concept is a recently patented system [5] that converts an existing nonductile steel brace into a buckling-restrained brace. The technique is made possible by a special type of thin Fiber Reinforced Polymer (FRP) laminates developed a few years ago by one of the authors [6]. The laminates are constructed with specially-designed equipment. Sheets of carbon or glass fabric up to 60-in. wide are saturated with resin and passed through a press that applies uniform heat and pressure to produce the laminate (Fig. 2). Depending on the type of fabric (glass or carbon), the laminates provide a tensile strength ranging from 60 to 155 ksi. Typical thickness of the laminates is 0.025 in., resulting in a flexible laminate that can be bent by hand (Fig. 2).
![Figure 1. BRB: (a) Schematic; (b) Load Deflection Comparison with CBF [4]](https://pilemedic.com/wp-content/uploads/2023/03/fig-1-buckling-restrained-braces-1024x360.jpg)
Since their introduction, the versatility of these laminates has led to be used in many structural rehabilitation applications worldwide, including bridge piers [7], submerged piles [8], cell phone towers [9], and pressure pipes in gas industry [10]. The thin laminates are flexible enough that can be wrapped around a brace to create a tube as small as 6-in. in diameter. A roll containing 200-feet of the laminates can be easily carried to anywhere in the building where the retrofit is required.
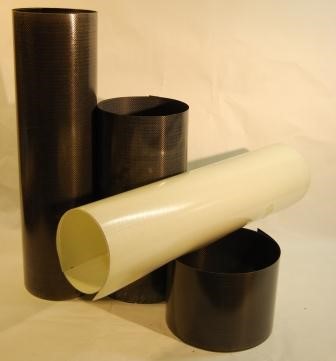
Retrofit Procedure
Due to space limitation, the retrofit steps are shown using the photos from a specimen that was tested as described in the following section. To ensure proper behavior as a BRB, a debonding agent should be used to prevent composite action between the brace and the concrete. In this case, duct tape was applied to the brace and it was coated with a bond-breaking material. Special plastic spacers were used along the edges of the brace to make sure the laminate does not come in contact with the brace (Fig. 3a). The 4-ft wide laminate is cut in a length equal to twice the perimeter of the tube to be made, plus 8 inches. An epoxy paste is mixed and applied to the laminate except the portion that will be directly facing the brace (Fig. 3b). The laminate is wrapped around the brace and bonded to itself to create a two-ply tube of desired diameter.
Because the epoxy has not cured yet, the size of the tube can be adjusted and temporarily held in the desired size using a few ratchet straps that are wrapped around the tube. The bottom of the tube is sealed, and the tube is filled with concrete. The next laminate is similarly installed, with an adequate overlap length that is epoxied to the first tube. This process is continued until the entire brace is retrofitted in 4-ft increments.
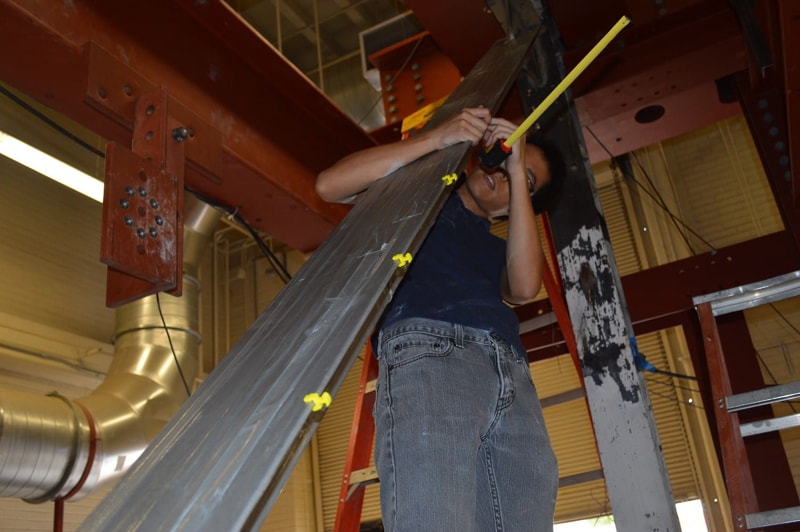
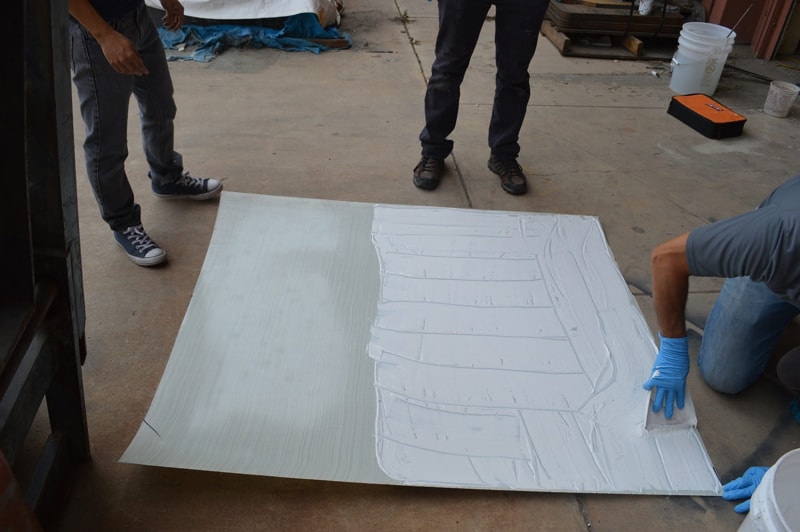
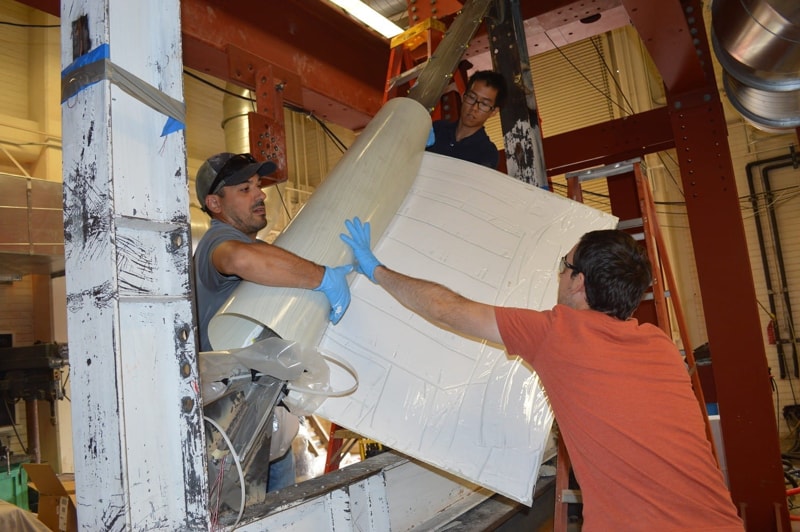
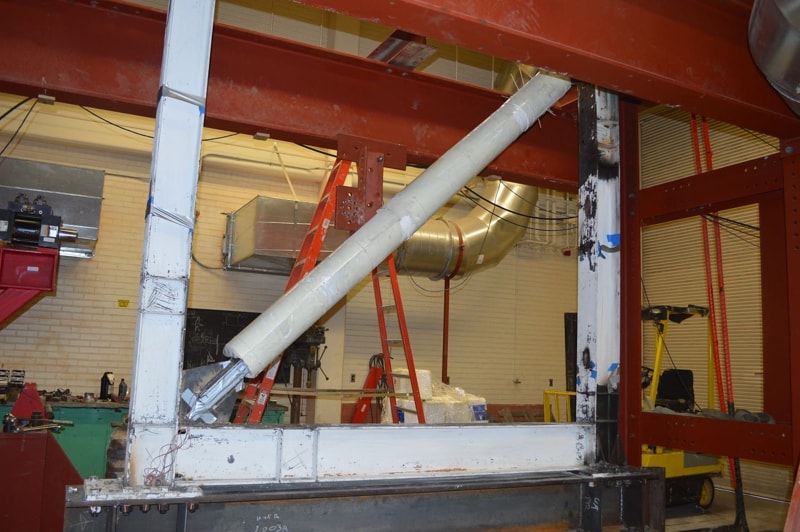
(b) apply epoxy, (c) wrap laminate around the brace to create a tube, and (d) fill the tube
with concrete.
The technique offers several unique advantages:
- It is applicable to both retrofit of existing frames and construction of new structures; the latter will reduce the cost of BRBs in new buildings that is currently controlled by few suppliers.
- The low-cost solution is very easy to implement in the field.
- Repairs require no major equipment and can be performed with a 2-man crew.
- Unique design is one-size-fits all, and eliminates costly custom-made products.
- Materials are lightweight and can be readily delivered to upper floors of a building using passenger elevators and stairways.
- Building safety is not compromised since the existing braces are not removed during the modifications.
Pilot Testing for Demonstration of Concept
A pilot test for BraceWrap proof of concept performed at the UA Laboratory. The test objective was to directly compare the performance of a CBF w/ and w/out the retrofit. The brace element was a welded double angle LL3½x3½x¼ with effective slenderness KL/r = 87.9. The BraceWrap retrofit (See Fig. 4c) used a 60ksi glass laminate formed into an 8¼” cylinder shell, held in place by plastic spacers (3D printed in-house), and filled with high-strength non-shrink quickset grout. The plies were held together using in-house epoxy paste. Surface preparation used household products: The ½” separation between angles was first filled with grout. The bond breaker was duct tape smeared with high temperature wax release. The grout, held in with a thin Polythene sheet, hardened in 3hr; the epoxy cured in 12hr. The total installation took two workers less than 3 hours. The materials cost for the BraceWrap® laminates, the epoxy paste, and the grout was less than $1000.
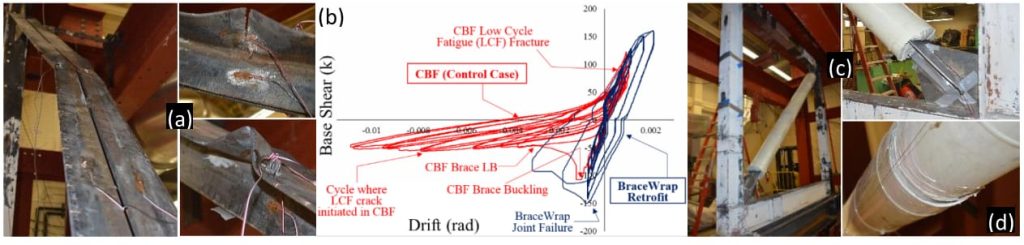
The unretrofitted brace incurred flexural-torsional buckling, leading to a rapid drop of load, followed by local buckling of the plastic hinge region, and after a few bucklingstraightening cycles, a low-cycle fatigue fracture through the entire outstanding flange of the hinge region (Fig. 4a). BraceWrap® successfully increased the buckling load of the brace by over 40%, significantly increased energy dissipation, and suppressed the local buckling and lowcycle fatigue failure of the brace (Fig. 4b). Just this improvement can make the difference between significant damage and modest damage in a CBF structure, or even prevent collapse for a highly vulnerable CBF.
However, lessons learned in the test provided insight into oversights in the installation process for the pilot test that are easily remedied, and should lead to significant further increases in strength and performance. Foremost was the inattention to the location of joints between the laminate sheets. For convenience, the sheets were placed from the bottom of the brace (with grout inserted in stages). As such, one of the joints was located within 2½” of the brace midspan. This joint failed (Fig. 4d), leading to the loss of load carrying capacity. It is believed that simply centering one of the sheets to straddle the mid-span would be enough to eliminate this failure. However, increasing the minimal laps (12” overlap) and epoxy used in this first trial are also parameters that warrant further investigation. It was clear from the test that the single sheet of laminate itself is strong enough to carry the hoop stresses; however, a second layer of staggered sheets over the joints is always possible at minimum extra cost. Finally, the desire to minimize the cross-section left the minimum grout cover 3/8” at the outstanding flanges. While it is not believed this led to the failure, the 8¼” diameter could easily be increased to provide more cover, and will be a design parameter.
It is noted that the bond breaker scheme fulfilled its objective, as the elastic stiffness of the retrofitted frame was similar to the original frame, and the core slipped +0.12/-0.09” on the brace. An additional identical specimen is currently in preparation where the above issues will be addressed. It is believed that by removing the overlap joints away from the midspan of the brace and/or increasing the number of wraps from 2 to 3 significant enhancement in the behavior of the retrofitted system will be observed. These modifications will have an insignificant impact on the cost of the proposed retrofit system.
Conclusions
This paper presents the undergoing development of a new cost-effective solution to convert an ordinary concentrically braced frame to a buckling restrained braced frame. The results of a pilot experimental study have been presented. Two welded double angle braces were tested, one as a control specimen and the other as a retrofitted frame. The experimental results indicate an increase of greater than 40% in the retrofitted specimen compared to the control specimen. However, the retrofitted specimen failed prematurely due to a design error by placing an overlapping joint at the midspan of the brace. An additional test is underway where the joint will be removed away from the midspan. It is anticipated that the performance of that specimen will be significantly improved compared to the first test.
The proposed retrofit system could be accomplished in 3 hours with a two-man crew and the materials cost were below $1000. The installation requires no heavy lifting equipment and can be easily carried out in locations where access is limited. These factors indicate that the propose system could have a significant value on reducing the seismic vulnerability and enhancing the safety of many existing structures.
References
- Fahnestock L, Hines E, Tremblay R, et. al.. Reserve Capacity & Implications for Seismic Collapse Prevention for Low-Ductility Braced Frames in Moderate Seismic Regions. Proceedings of the 10th NCEE, Alaska, 2014.
- Fleischman R, Restrepo J, Pampanin S, Maffei J, K.Seeber K, and Zahn F. Damage Evaluations of Precast Structures in 2010-11 Canterbury Earthquake. Earthquake Spectra, 2014; 30(1):277-306.
- Peterson M, Moschetti M, Powers P, Mueller C, Luco N, et.al.. Documentation for the 2014 Update of the United States National Seismic Hazard Maps. USGS, 2014.
- Star Seismic. 6300 N Sagewood Dr, Park City, UT 84098. http://www.starseismic.net/
- Ehsani, M. 2017. Buckling Reinforcement for Structural Members, US Patent No. 9,719,255, U.S. Patent and Trademark Office, Alexandra, VA, 15 pp.
- Ehsani, M. FRP Super Laminates: Transforming the Future of Repair and Retrofit with FRP. Concrete International 2010; ACI, 32(03): 49-53.
- Ehsani, M., and Croarkin M. A Novel Solution for Restoration of Deteriorated Piles. Government Engineering, March-April 2011, 14-15.
- Ehsani M, Day S, and White T. Repair of ASR-Damaged Piles in a Crocodile-Infested River.” Concrete International 2017; American Concrete Institute, Detroit, MI, 39(4): 61-65.
- Ehsani M, and Rimmele RJ. Structural Challenge Facing the Wireless Communications Industry – A New FRP Solution for Strengthening Concrete Telecommunication Towers. Structure Magazine 2016; 23(12):22-24
- Carbone M, Ehsani M, and Ragula G. Winter Wonderland Does Wonders to CIPP Renewal of a High-Pressure Gas Main in New Jersey, North American Society for Trenchless Technology (NASTT) No Dig Show 2012; Nashville, TN, Paper E-1-01, 10 pp.